Prof. Sanjeev Dhurandhar is a physicist and Emeritus Professor at the Inter-University Centre for Astronomy and Astrophysics (IUCAA), Pune. He has made seminal contributions to gravitational-wave physics, particularly to the data analysis techniques for the detection of gravitational waves. Presently, he also serves as the Science Advisor to the Indian Initiative in Gravitational-Wave Observations (IndIGO) Consortium Council.
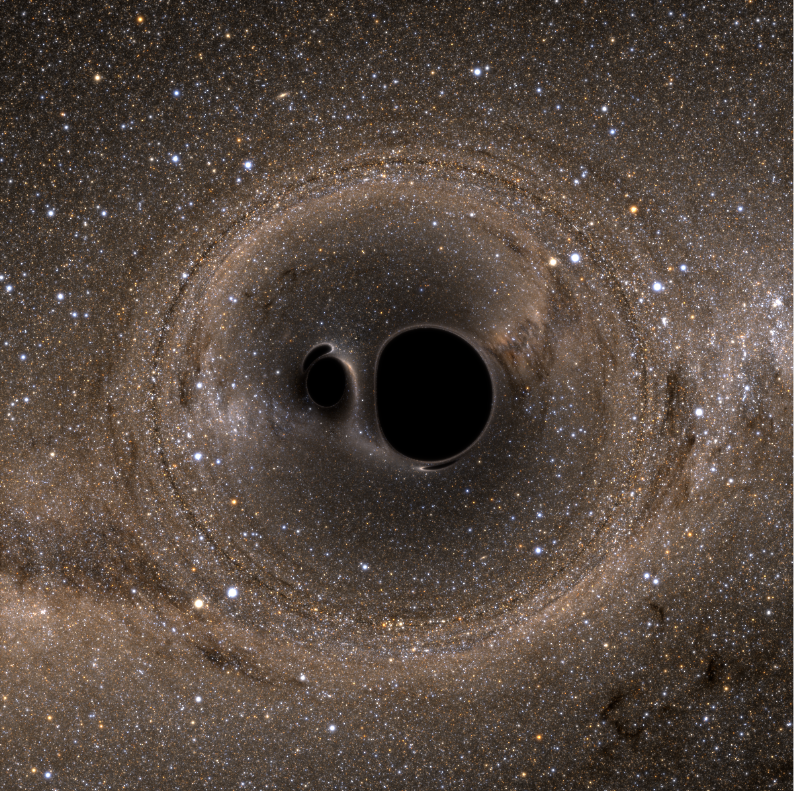
Gravitational-wave detections have opened a new window to the universe in astronomy. The first window in astronomy was opened by Galileo 400 years ago when he pointed his telescope at Jupiter and observed its moons. Then, radio astronomy started 70 years ago and immediately made many discoveries like the CMB, pulsars, radio galaxies, quasars, etc.
1. What got you motivated into science as a student? And as a young researcher what got you interested particularly in general relativity and gravitational wave physics?
Science was always my primary interest, so nothing got me motivated into science. Kids of my age were more interested in other things, like playing and socialising. However, I was always curious to find out new things and try them out and understand them. I was always interested in science since birth (laughs).
As for the interest in gravitational waves, things sort of led to it, in a way. I was interested in both mathematics and physics in my childhood. I liked engineering as well; I used to build electromagnets and other things like that in childhood. But I was equally interested in mathematics. Once I was trying to trisect an angle with a compass and a ruler, and I'm happy my teachers didn't know that it can't be done; it kept my curiosity and interest in mathematics alive. Back then, general relativity was taught as a mathematics course. Since I had taken mathematics, I got to learn general relativity and I found it very interesting.
I did my PhD on black holes and also worked for a year with Prof. Govind Swarup at the GMRT (Giant Metrewave Radio Telescope). In 1986, I heard a talk on gravitational-wave detectors by Prof. Kip Thorne (Nobel Laureate in Physics, 2017). That was the first time. Later, I talked to Prof. Bernard Schutz who told me that it (the gravitational-wave detector) involves general relativity as well as antennas. I was interested in both and took up gravitational-wave physics. I realised that it was a major challenge. I did not care about success at that time and well, it (the theory) did succeed.
2. What differences do you see in the gravitational-wave physics community between when you started and now? What are the revolutions/major insights you think the recent detections have resulted in?
Well, there was no gravitational-wave community back then! There were a few prototype detectors; prototype, because they couldn't detect anything. There were a few small detectors of size of the order of tens of metres.
Prof. Ronald Drever was very instrumental in coming up with innovative ideas for this project. He would come up with a new idea for the detector everyday. He would have been one of the Nobel Prize recipients had he not died this March (2017). At this time (Prof. Bernard) Schutz and (Prof. Kip) Thorne were concerned about how we could analyse data from gravitational wave detectors and what sources could be detected. It was Thorne who realised that compact coalescing binaries are the most promising sources for laser interferometric detectors and all the events detected so far have been of this type. Initially, very few groups were working in this area. As for the recent detections, the findings about the masses of black holes were surprising. Earlier, we used to think that stellar mass black holes are about 10 solar masses, but the first detection itself were black holes of about 30 solar masses. This was an addition to our knowledge. And now people have to come up with mechanisms to explain how such big black holes can be formed. This was also the first direct detection of gravitational waves, black holes, and black hole binaries. Also, these events are completely consistent with the general theory of relativity, which add one more feather to Einstein's cap!
3. What are the questions you are particularly hopeful about and wish to get solved in the field?
Gravitational-wave detections have opened a new window to the universe in astronomy. The first window in astronomy was opened by Galileo 400 years ago when he pointed his telescope at Jupiter and observed its moons. Then, radio astronomy started 70 years ago and immediately made many discoveries like the CMB (Cosmic Microwave Background), pulsars, radio galaxies, quasars, which couldn't have been made by optical astronomy. Then we have infrared astronomy, which has shown us exoplanets and other stellar systems like ours. So as soon as a new window opens, it enables us to discover new things in our universe. It complements our previous knowledge. I think gravitational-wave astronomy will do the same thing. The recent neutron star coalescence event has told us so many things. For example, it has confirmed that the speed of gravitational waves is not different from the electromagnetic waves, as the gamma rays were also detected around the same time after the merger.
4. How vital a role is LIGO-India going to play in the coming decades in your view? What role do you see young students in science playing in the project, if any?
Speaking in terms of astronomy, LIGO-India has got a strategic location. Combined with the other detectors in the US and Europe, this will give a much longer baseline. These detectors are omni-directional. So a longer baseline will help us determine the location of the event more precisely. These detectors play a similar role to gravitational waves as dipoles earlier did to electromagnetic waves. Further apart the detectors are, the better it is to determine location of transient sources. Also, the orientation of LIGO-India will be different from the rest (of the observatories), which will help in getting more information about the polarisation of gravitational waves.The polarisation contains information about the orientation of the source. In case of the binary (neutron stars or black holes) it is the angle of inclination of the orbit. LIGO-India will improve the determination of location by one or two orders of magnitude compared to just the LIGO detectors.
Also, being in India, it will attract significantly more advanced technology. Young students in science will have a big role to play in this. We will need good engineers and physicists. The problems encountered here will be different, and we will need creative minds to solve them. Our aim is to make the detectors even better than the present ones in terms of technology and other aspects.
5. The Laser Interferometer Space Antenna (LISA) is a very ambitious upcoming project. You have yourself been involved in calculations related to the time-delay interferometry for LISA. Can you elaborate on the work? Also, what are the major expectations from LISA?
It is difficult to detect waves with frequency below 10 Hz using ground-based detectors. Variations in local gravitational fields shake the mirrors - this is called Newtonian noise and is difficult to eliminate. Moreover, to detect some specific objects like supermassive black holes or things falling into massive black holes, we need to go below the current bandwidth, to the millihertz (mHz) range. Space detectors will allow us to make such detections. Just like radio astronomy complements optical astronomy, space detectors will complement ground-based detectors and will help us find new sources in a different frequency band.
However, LISA has interferometers with unequal arm lengths as it is difficult to keep the spacecrafts equidistant. (LISA will have a constellation of three spacecrafts, arranged in an equilateral triangle with sides 5 million km long, orbiting the sun in a manner similar to earth.) But laser frequency noise is a problem for LISA because it is an unequal arm interferometer - it is impossible to make the arms equal in length. This noise is many orders of magnitude more than other noises. In ground-based detectors, this noise is common to both arms which are equal in length and hence, it cancels out. In LISA we have six different data streams between the three spacecrafts, and we must build the interferometer in software. We must appropriately delay the data streams so that the laser frequency noise is cancelled. This is called time delay interferometry. I recognised that this problem can be mapped to a problem in algebraic geometry, more specifically in commutative algebra. This problem has already been solved by mathematicians. We could then apply their results to the problem in LISA.
The interview of Prof. Sanjeev Dhurandhar was done by Onkar Sadekar and Anwesha Maharana for Aakashganga, the Astronomy and Astrophysics club run by the students at the Indian Institute of Science Education and Research, Pune, India. This interview originally appeared in Dhruv - the newsletter of Aakashganga. Onkar Sadekar and Anwesha Maharana are pursuing undergraduate degree at IISER Pune.